June 6, 2024
More sustainable and cost-efficient Na-ion batteries are poised to make an impact for large- and grid-scale energy storage applications
While Lithium-ion (Li-ion) batteries have become ubiquitous over the last three decades — powering everything from personal electronics to electric vehicles to grid-scale applications — the search for next-generation battery chemistries is gaining speed. Fueled by sustainability and environmental challenges, supply chain volatility, and safety concerns, companies are actively exploring a variety of alternatives like solid-state batteries (SSBs) and silicon-anode technologies, with product launches planned for smart phones, e-mobility devices, and EVs in the coming years.
Sodium-ion (Na-ion) batteries are another potential disruptor to the Li-ion market, projected to outpace both SSBs and silicon-anode batteries over the next decade, reaching nearly $5 billion by 2032 through rapid development around the world. Chinese battery mainstay CATL and U.K. startup Faradion (since acquired by Reliance Industries) are among the companies leading commercial sales of Na-ion battery products, and the first Na-ion battery plant in the U.S., operated by Natron Energy, is scheduled to begin deliveries for grid-scale Na-ion battery products this summer.
However, the race to commercialization is happening in parallel with ongoing research and development efforts — underscoring the need to scrutinize the performance of Na-ion batteries. Similar to Li-ion batteries, Na-ion technologies are likely to face unexpected challenges for battery manufacturers and their end users, ranging from grid-scale operators to OEMs to tech companies, who will be faced with new safety, performance, and product/system integration questions. These issues could be compounded by the accelerated pace at which Na-ion batteries are being introduced into the market, as stakeholders are only at the beginning of gaining the critical real-world insights needed to help ensure reliability at scale.
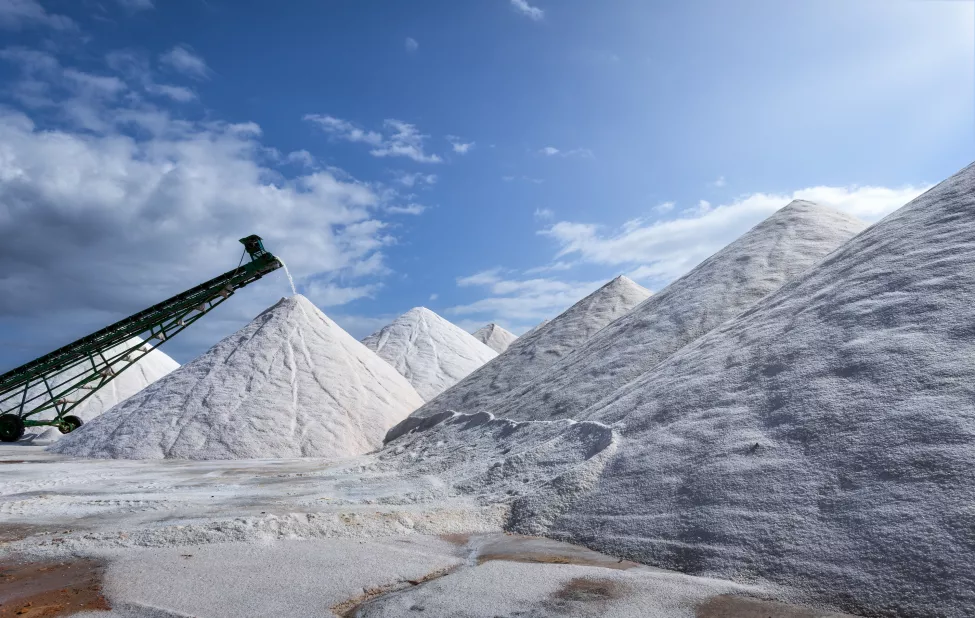
Increasing demand meets broader, more sustainable availability
Market research estimates that U.S. data center demand will reach 35 gigawatts annually by 2030 — double the demand from 2022. Similarly, grid-scale energy storage is projected to surpass 400 gigawatts in the same time frame — a tenfold increase over 2023 installations. Meeting the rising demand for these two applications alone will require a battery chemistry that is more sustainable and cost-effective in the long run.
As the sixth most abundant element on Earth, approximately 1,000 times more abundant than lithium, sodium offers a far more available alternative. Na-ion batteries are primarily composed of sodium, aluminum, and mixtures of other materials, which, at scale, could amount to an estimated 25-30% reduction in material costs compared to lithium iron phosphate (LFP) batteries — the type of Li-ion battery most commonly used in utility-scale applications and increasingly in EVs, requiring not only lithium but cobalt and nickel as well. Given that lithium stores could be exhausted by the end of the 21st century and that Na-ion battery cells are estimated at one-third the price of their Li-ion counterparts, Na-ion batteries present an attractive solution to some of the ongoing challenges facing battery manufacturers.
Though they do require materials that demand global sourcing, Na-ion batteries could also shift the concentration of battery manufacturing in the world, allowing countries previously dependent on complex supply chains to produce batteries at new volumes. The U.S. holds the majority of the world's natural soda ash — otherwise known as sodium carbonate, the primary industrial source of sodium — which could further accelerate the development and manufacturing of Na-ion technologies in North America.
"Thinking through the lessons learned over the past 30 years of Li-ion battery development can help stakeholders get ahead of unexpected challenges for Na-ion batteries"
Current limitations and opportunities for growth and innovation
In addition to cost and resource advantages, Na-ion batteries are attractive for their high thermal stability and potential lower flammability. These important properties allow for higher operating temperatures and lower voltages relative to lithium batteries, meaning they offer greater potential safety benefits.
The key drawback to Na-ion technologies is lower energy density. Their current average gravimetric energy density is estimated at 150 watt-hours per kilogram compared to an average of 265 for Li-ion, although Na-ion are projected to break the 200 watt-hours per kilogram ceiling in the near future. Sodium ions are also larger and heavier than lithium ions, which means they occupy more space and require more material to store the same amount of charge, resulting in a comparatively limited amount of energy per unit mass or volume of the battery. This offers safety and performance benefits for compact, inexpensive, and range-limited EV fleets as well as data centers and grid-scale applications, where the power demand on the battery supply is largely consistent and the consequences of thermal runaways near communities and inside large buildings may be reduced. By contrast, Na-ion technologies for sleek, light-weight electronics and long-range driving applications that require higher voltages may be further afield.
To expand beyond these limitations, particularly at scale, improving the conductivity and electrochemical stability of Na-ion batteries is a key focal point of research and development, as well as experimenting with cathode selection and anode material composition to increase their cell capacity and, ultimately, energy density.
Cathode materials, including layered oxides, polyanionic compounds, and Prussian blue analogs (PBAs), along with anodes including hard carbon or ones that include tin, antimony, bimetallic alloys, or bismuth-based transition metal chalcogenides (TMDs), have produced an array of potential solutions to enhance Na-ion energy density and electrochemical performance — each, however, with their own accompanying drawbacks and challenges. For example, PBA's can release toxic gasses at high temperatures (above 200 C) as a result of thermal runaway. On the other hand, layered oxide cathodes may not benefit from the same improvements in thermal stability as polyanionic and cyanate based cathodes, and some Na-ion cells still use flammable organic electrolytes similar to those in Li-ion batteries.
For product designers, carefully assessing compositional chemistry opportunities against the risks of particular material use, as well as the energy requirements of the product, its failure modes, and foreseeable use and misuse scenarios, will be critical to addressing safety — as will performing rigorous cell and pack-level testing and selecting the right combination of electrode materials. These choices are best made with respect for the specific application and intended use case. Design and manufacturing principles for Li-ion batteries may not be relevant to Na-ion batteries. For example, aluminum current collectors should be used for sodium negative electrodes, not just for cost savings, but for electrochemical stability at low voltages.
Understanding the performance of a battery across its lifecycle is also essential. Advanced analytical techniques such as accelerating rate calorimetry (ARC), fractional thermal runaway calorimetry (FTRC), and X-ray computed tomography (CT) can support the evaluation of Na-ion failure modes, thermal runaway scenarios, and the risks presented by variations in cathode and anode electrochemistry.
Preparing for the future of Na-ion demand, development, and optimization
Innovative, cost-effective, and more sustainable solutions like Na-ion battery technology are gaining momentum, presenting manufacturers, utilities, OEMs, and potentially electronics companies with new opportunities to meet future battery demands — especially in filling the role of batteries at the largest scale that power households, communities, and critical telecommunications and data storage systems.
However, with the introduction of any new technology comes some risk. Thinking through the lessons learned over the past 30 years of Li-ion battery development can help stakeholders get ahead of unexpected challenges for Na-ion batteries. Ongoing electrode research and product integrations will benefit from rigorous quality, risk, and safety assessments, helping ensure reliable battery performance in real-world applications.
Importantly, though the use of sodium offers considerable environmental benefits over lithium, the full supply chain for the many materials used in Na-ion batteries remains in its infancy. Manufacturers and product developers will need to carefully assess and evaluate component integrations, sourcing options, and supply chain vulnerabilities to ensure quality and reliability. Likewise, defining risks specific to the manufacture, transport, and storage of Na-ion batteries and implementing processes for post-market surveillance and iterative improvements will be necessary to continuing on the path to widespread commercialization.
What Can We Help You Solve?
Exponent has been at the forefront of Li-ion battery development for three decades, pushing beyond standardized tests to improve battery performance in complete, integrated products. With multidisciplinary expertise in energy storage, including Na-ion technologies, we help clients evaluate battery compatibility, safety, and reliability through design reviews, risk and safety assessments, and failure analysis.

Batteries & Energy Storage
Supercharge performance, reliability, and safety across all stages of the battery and energy storage product lifecycle.
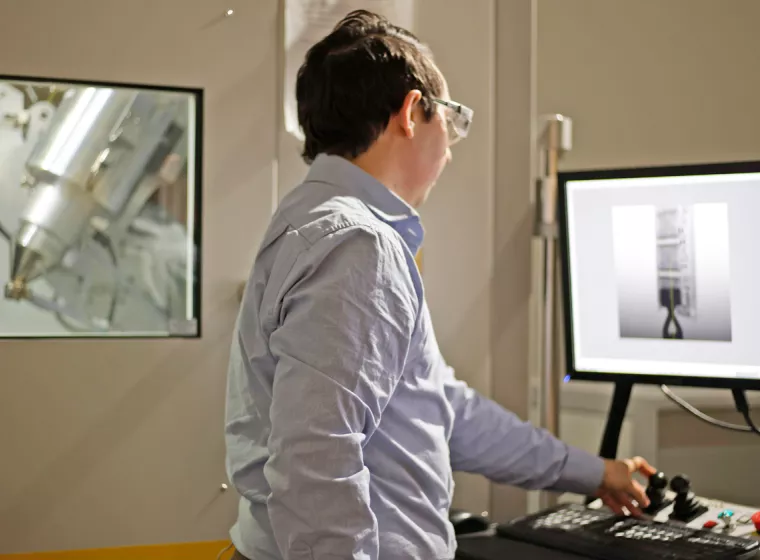
Custom Battery Testing
Custom product safety, performance, and industry certification battery testing services.
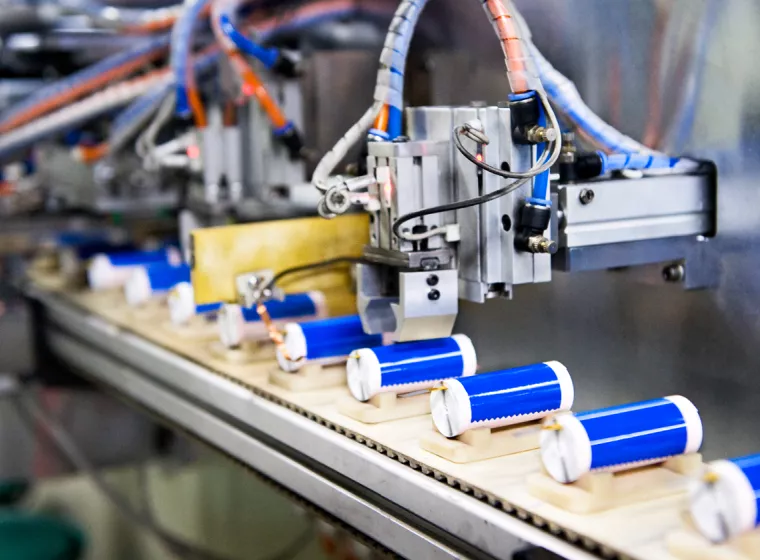
Battery Supply Chain Services
End-to-end battery supply chain support including evaluating potential manufacturer partners and suppliers
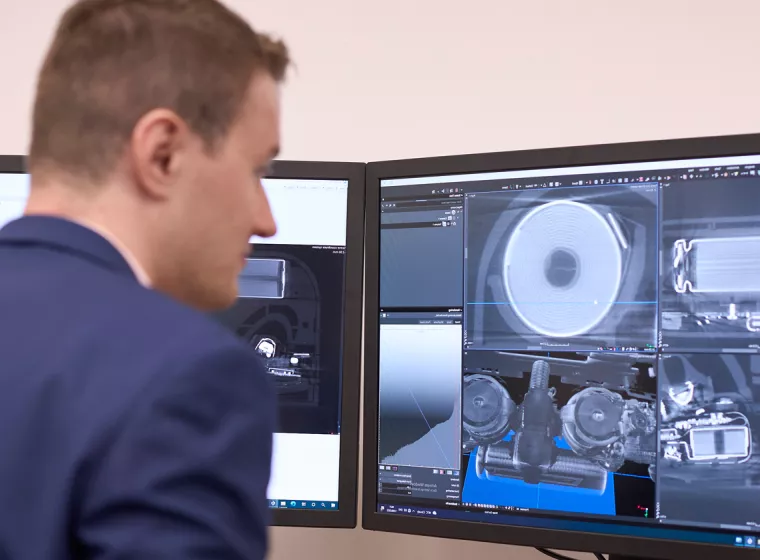
Battery Design Review, Quality & Safety Assessments
State-of-the-art battery design, performance, quality, and safety assessments to support manufacturers' requirements and industry standards.
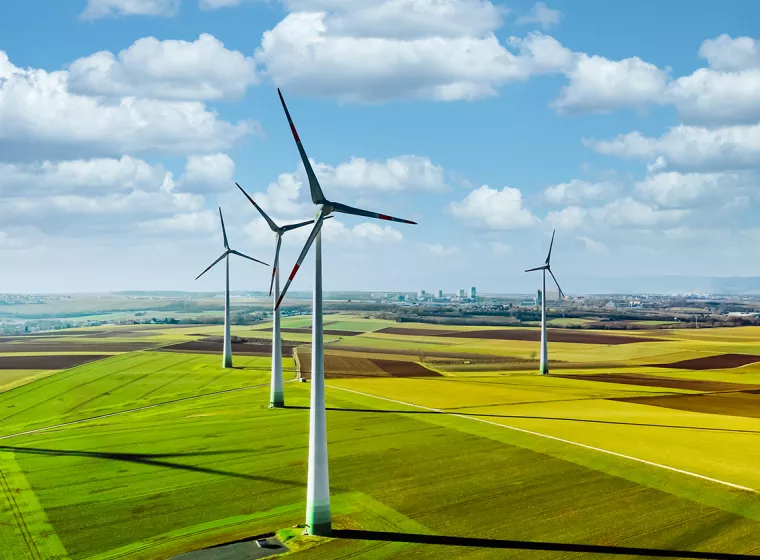
Power & Energy
Multidisciplinary support for high-power energy systems of all sizes in a variety of applications.
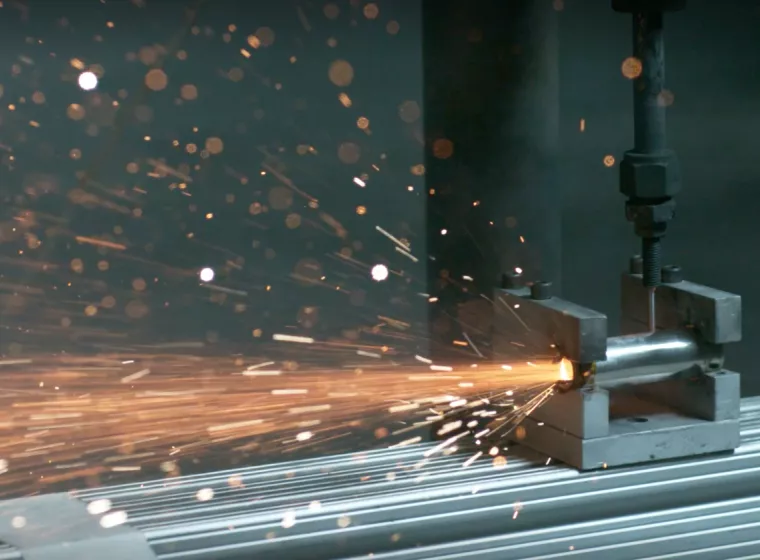
Battery Failure Analysis & Investigation
Comprehensive battery failure analysis to determine the root causes of failures and identify opportunities for mitigation.
Insights
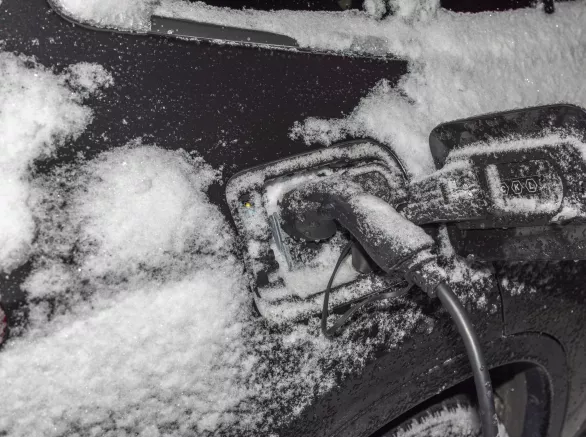