February 7, 2025
Approaches to battery energy storage system planning, construction, integration, and safety
Since 2010, utilities in the U.S. have added almost 20 GW of battery storage to the grid. Battery energy storage systems (BESS) are being used across the country to store power from renewable energy sources, like wind and solar, and as power backup systems for critical infrastructure, like hospitals, factories, and municipal facilities. According to the U.S. Energy Information Administration, operators added 5 GW of BESS to the U.S. power grid in the first seven months of 2024 and dozens more systems are awaiting approval.
Despite this surge of domestic utilities integrating more BESS into their grids than ever before, few standards exist to guide their deployment or account for the variety of available systems, components, and interconnection specificities. There are, however, best practices for planning, building, integrating, and managing BESS that can make the process faster, safer, and more efficient for both operators and the communities they serve.
What are BESS?
BESS are large, stationary batteries used to store energy from the grid, often from renewable energy sources. They provide power to the grid during power outages or fluctuations and during peak demand periods. They are also used to provide power when renewable energy sources cannot — at night or when winds are low.
There are a variety of BESS solutions available, from lithium-ion battery stacks to cutting-edge sodium-ion cells to more traditional lead-acid systems. There is no homogenous way of storing large amounts of energy, with utilities often building custom solutions or contracting with renewable energy providers to develop BESS. Despite the wide array of options, all systems include several key components:
- Cells, modules, and racks (i.e., the battery pack): The actual batteries that store energy, including the battery cells themselves, modules of those cells, and racks of modules.
- Battery management system (BMS): The electronic components that monitor battery voltage, current, and temperature and protect against overcharge, overdischarge, overcurrent, overheating, etc.
- Power conversion system (PCS): The system that converts direct current energy stored in the batteries to alternating current for the grid and vice versa.
- Thermal management system: Active cooling equipment like radiators and fans to maintain battery temperature during charging, discharging, and storage.
- Energy management system (EMS): The system of electronics used to direct energy into and from BESS.
- Safety controls: Various equipment meant to protect personnel and to contain and mitigate fires caused by battery failures.
BESS manufacturers are as varied as BESS themselves, each with their own unique approach to building these large systems. While they all adhere to certain battery standards (different from the lack of standards governing BESS deployment by utilities), the BMS, PCS, EMS, and safety features in any given BESS may differ significantly.
"High-profile BESS fires like the Surprise, Arizona fire show that large quantities of li-ion batteries can and do experience thermal runaway if they are damaged or a fault occurs in the system."
Planning for BESS
As with any complex utility project, BESS deployment requires thorough planning. One of the first steps in planning is regulatory review. BESS, as a new form of utility project, do not yet have their own set of detailed regulations to help guide manufacturers and utilities. However, many National Fire Protection Association (NFPA) and International Fire Code (IFC) regulations provide some guidance. These include regulations around fire detection and protection, installation, emergency procedures, ventilation, thermal management, lightning protection, and signage. These regulations do not necessarily apply to BESS specifically but to all types of energy storage systems. Other general codes and regulations for power equipment safety also apply to BESS, like codes from the International Code Counsel (ICC) and National Electric Safety Code (NESC).
Adoption of these standards and codes, however, is mostly voluntary and depends on the location of the energy storage system. Some states, like California and New York, have unique safety codes and standards related to energy storage systems that can be applied to BESS. This means that safety codes and standards for BESS are often not adopted consistently and can be misinterpreted. Utilities should check with local authorities and government bodies to ensure the BESS they deploy meets local safety and environmental standards. If not, they could face fines or, worse, experience dangerous BESS malfunctions caused by improper installation and operation.
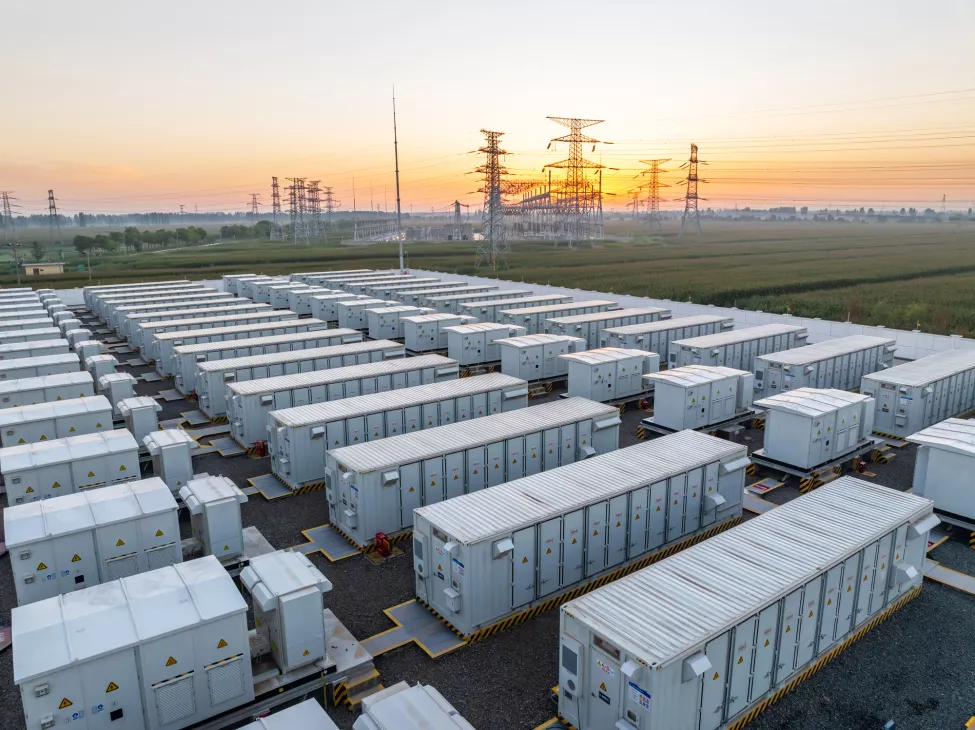
Assessing and managing BESS risks and hazards
Risk assessments are another early step utilities can take when planning for BESS. High-profile BESS fires like the Surprise, Arizona fire show that large quantities of Li-ion batteries can and do experience thermal runaway if they are damaged or a fault occurs in the system. In the case of the Surprise fire, firefighters had difficulty extinguishing the blaze and the BESS eventually exploded, injuring four responders and releasing toxic substances into the surrounding environment. One of Exponent's findings in this investigation revealed that there was insufficient thermal shielding between battery cells, which led to the thermal runaway and explosion. Emergency response teams were also found to have had inadequate training to deal with Li-ion fires.
Any organization assembling or installing BESS should be aware of their associated hazards and prepare to identify and mitigate them. In general, there are three primary hazards and failure modes for BESS:
- Electrical hazards — These include shock and burn hazards to personnel and operators, along with electrical equipment failures that can either disable the BESS or lead to thermal runaway and fire in the battery cells. Shock risks can be mitigated with safety equipment like enclosures and proper earth grounding. Thermal runaway hazards can often be mitigated through physical barriers between cells and adequate battery monitoring and safety systems like fire suppression systems.
- Mechanical hazards — These include any mechanical components that can harm operators like switches and other moving parts. They also include damage to battery cells or other systems that can cause thermal runaway, fires, or toxic conditions. Another potential mechanical hazard is the explosion of the BESS container, which is often made of metal, when filled with combustible gases from failing batteries. These deflagration/explosion risks can be mitigated through venting and other safety systems to evacuate or mitigate the gases during a failure event, preventing the dispersion of dangerous pieces of metal. Many mechanical hazards can be addressed with detailed engineering and planning, as well as thorough safety review of plans before construction begins.
- Environmental hazards — Environmental hazards like earthquakes, storms, freezing temperatures, heat waves, and wildfires can all damage BESS installations. Heavy rain, flooding, and even salt fog near oceans can damage BESS containers. Systems can be strengthened against environmental hazards structurally by supports that factor in weather and climate change-related hazards, reinforced seals, etc.
Failure modes and effects analysis (FMEA) is an effective approach to identifying hazards and risks for BESS. This systematic method is usually performed during the design stage to identify potential failure modes in a system and their effects on the system. In the FMEA process, hazards and failure modes and their effects are thoroughly defined and documented, along with mitigation strategies (see Fig. 1).
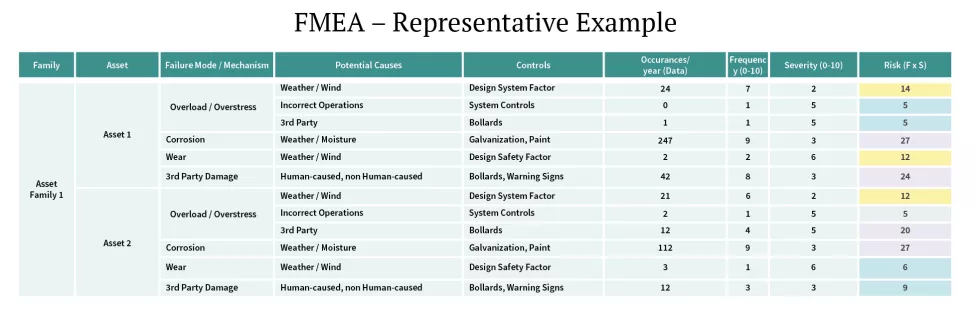
Challenges for responding to BESS fires
In the event of a BESS thermal runaway or fire, it's important for operators to be aware of their unique hazards. During BESS fires, hazardous substances may be released in the form of airborne gases and smoke particulates or entrained in firefighting water. These substances, which include acids like hydrofluoric acid; inorganic elements such as lithium, cobalt, and nickel; and organic substances such as short-chain per- and polyfluoroalkyl substances (PFAS), may pose a threat to fire response personnel, as well as to nearby people, animals, or other ecological receptors.
Utilities that plan for potential failures from the very beginning of BESS construction can minimize the environmental impacts of a fire. For example, many BESS installations are built on gravel catches that can contain runoff from firefighting water and help divert toxic substances.
Connecting BESS to the grid
Performing thorough risk assessments for BESS can help expedite system deployment, but there are many more hurdles to overcome in connecting BESS to power grids. According to a Lawrence Berkeley National Laboratory study, developers and stakeholders of BESS projects wait in interconnection queues for an average of four years or more. These queues are often packed with project proposals awaiting approval from regional energy transmission authorities or utilities. Obtaining approval to connect BESS to existing grids can be a long process involving many technical, environmental, and safety concerns.
- Technical Compatibility: Once an energy storage project is approved, it can require millions of dollars in power infrastructure upgrades to bring online. Existing grids were not designed to accommodate large-scale BESS, requiring significant time, investment, and modifications to ensure compatibility and efficiency. Assessing the full scope of development that might be needed through a grid integration study at the time of a proposal not only has the potential to streamline wait times but can help stakeholders avoid years of queuing only to find the true cost of interconnection isn't feasible.
- Grid Stability and Management: Managing the flow of electricity with the addition of storage systems requires advanced control systems to balance supply and demand, prevent overloads, and maintain grid stability. Mismanagement, especially over time, can increase the chance of failure and reduce operational outputs and the lifespan of BESS, amounting to lost time and money for utilities.
- Operations and Maintenance: Ensuring the reliability and longevity of storage systems requires ongoing maintenance and monitoring. This can be resource-intensive and requires specialized knowledge and skills. Neglecting this maintenance and proper operation can lead to reduced BESS lifespan and efficiency, or even catastrophic failure. Diagnostic data, which many BESS facilities generate large amounts of but don't systematically utilize, can offer early clues as to signs of a pending failure.
- Environmental and Social Impact: The installation of large-scale storage systems can have environmental and social impacts, such as land use concerns and community acceptance. Addressing these issues is crucial for successful integration.
- Interoperability: Different storage technologies and systems need to work seamlessly with each other and with the existing grid infrastructure. Achieving this interoperability can be technically challenging.
- Cybersecurity: Cybersecurity becomes a critical concern with the increased digitalization of grid systems. Protecting storage systems from cyber threats is essential to maintain the security and reliability of the grid.
More renewables, more BESS
Since energy storage is key to a solar and wind-powered future, BESS will be a critical part of the renewable energy transition. Utilities will continue to add BESS to the grid to supplement renewable energy sources and to provide backup power for emergency situations. As more systems are installed, more standards and best practices will emerge to help streamline the process. In the meantime, utilities and other BESS operators can mitigate the risks of installing and operating BESS with thorough planning and risk analysis.
What Can We Help You Solve?
With decades of experience working with batteries and energy storage systems and the utilities industry, Exponent's multidisciplinary experts provide total product lifecycle support for BESS. Our consultants offer risk assessments, compliance reviews, gap analyses, design reviews, vendor and supply chain evaluations, and bespoke testing that goes beyond the standards.

Batteries & Energy Storage
Supercharge performance, reliability, and safety across all stages of the battery and energy storage product lifecycle.
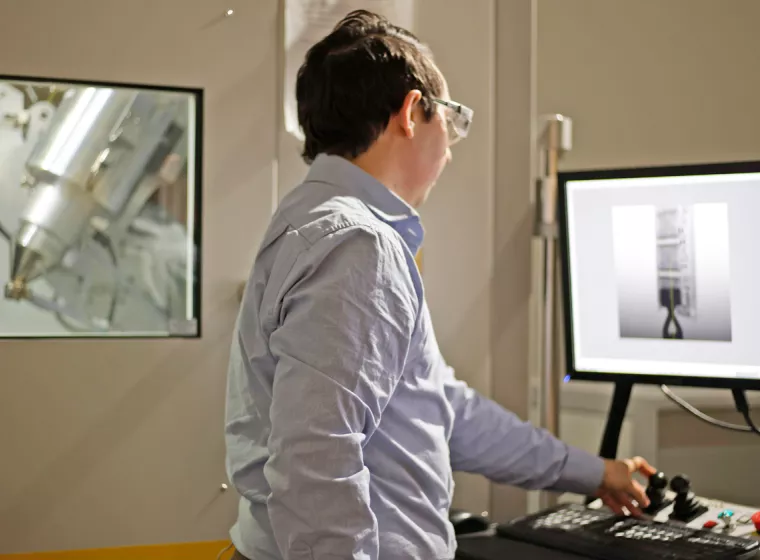
Custom Battery Testing
Custom product safety, performance, and industry certification battery testing services.
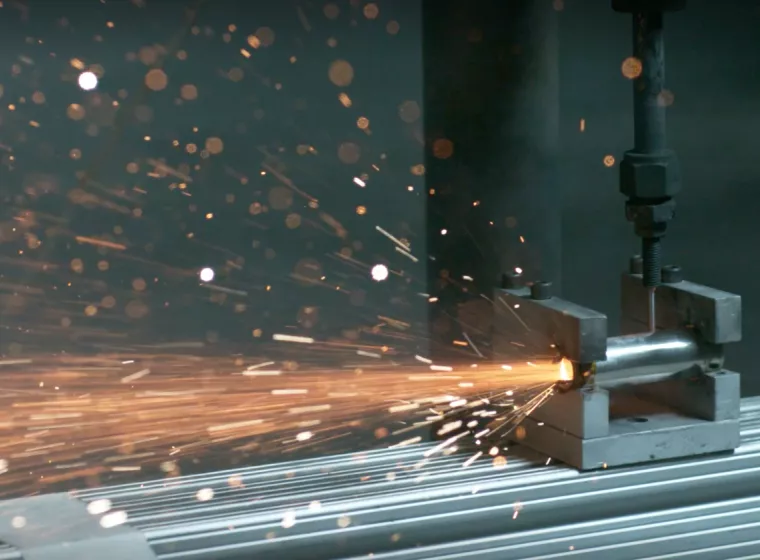
Battery Failure Analysis & Investigation
Comprehensive battery failure analysis to determine the root causes of failures and identify opportunities for mitigation.
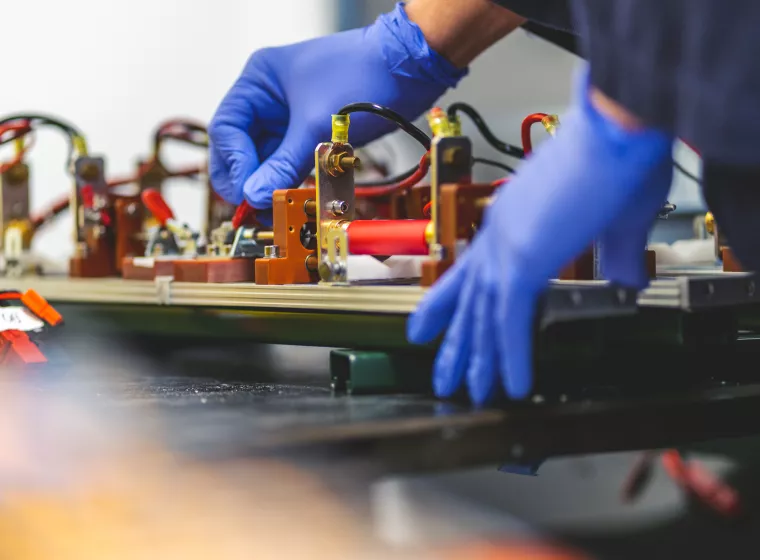
Battery Risk Assessments & Corrective Action
Li-ion battery risk assessment and corrective action services, including cost-effective tools for long-term monitoring.
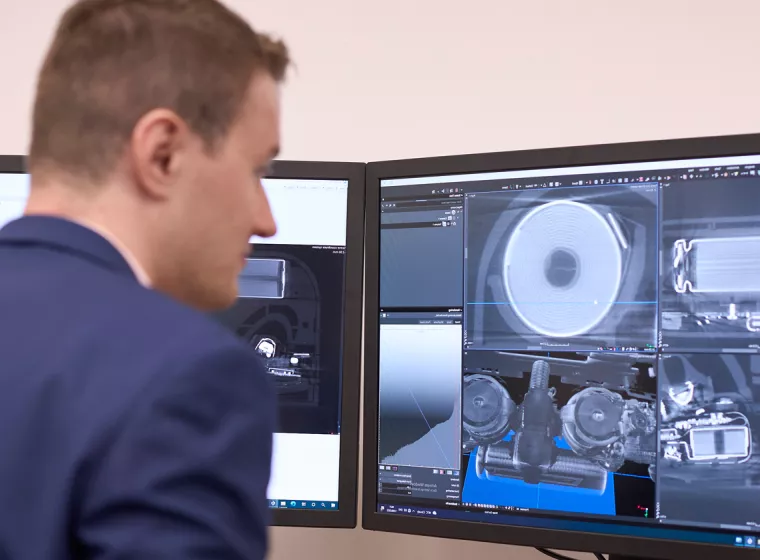
Battery Design Review, Quality & Safety Assessments
State-of-the-art battery design, performance, quality, and safety assessments to support manufacturers' requirements and industry standards.
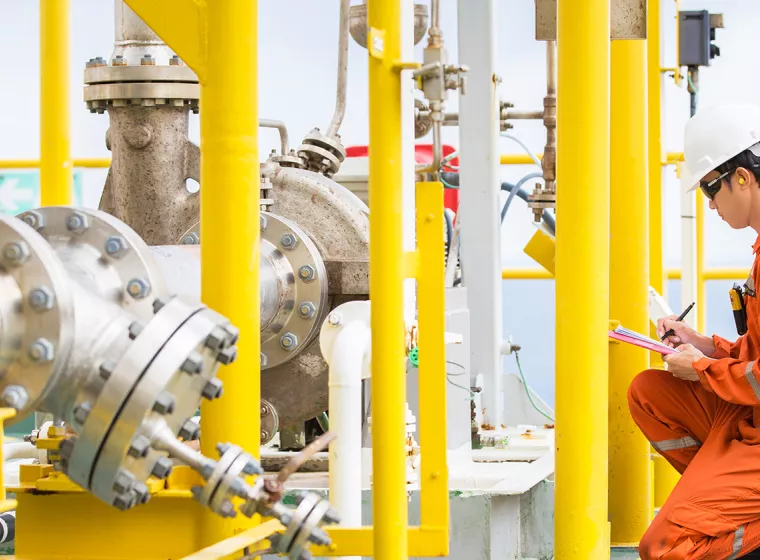
Metallurgical & Corrosion Engineering
Valuable insights for your most formidable metallurgical and corrosion engineering challenges.